I can confidently say that the usage of various types of lithium-ion batteries has created a huge impact on our daily life. The first thing that would strike me when I think about anything that is wireless and requires electrical energy is batteries. Specifically lithium-ion batteries.
In recent times, Lithium-based batteries had found their way through the automotive industry as well. Compared to the former wireless space, the mobility space has a lot of challenges. The main ones are storage capacity and safety. With alterations in the battery chemistry, we can find a battery that can cater for our requirements.
This is exactly what I will be discussing here in this blog. Let’s start with the contribution made by some popular elements for the performance/Safety of the battery.
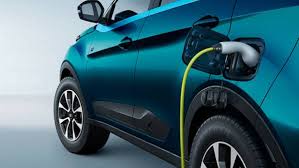
Before I start, let me introduce a couple of basic terms which will be useful henceforth.
Specific energy is the amount of energy you can pack per kilogram. It is measured in Megajoules per kilogram (MJ/kg) or watt-hour per kilogram (Wh/kg). In simple words, more is the specific energy lighter the battery gets. When you measure the same with volume, we call it energy density. More the energy density, the lesser the space the battery occupies. These two factors are pivotal to an electric vehicle.
The cathode is the positive electrode and the anode is the negative electrode in an electrochemical cell (battery). Whereas it is the opposite when it is an electrolytic cell (that is when the battery is charging). Knowing this, let’s get started!
Ohh Wait!! Have you checked out my previous blog on batteries used in EVs? if not please check it out by clicking here
Table of Contents
Elements and their role in Lithium-ion batteries
1. Lithium
Lithium is the smallest and lightest element in the universe. And of course, it is a metal. This is the vital property why Lithium-based batteries are very successful when we consider range. As the specific energy and energy density are very promising.
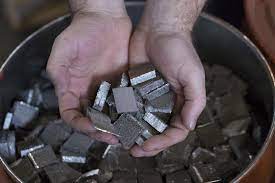
Because of its small size, it has very less internal resistance and hence the terminal voltage of these batteries is higher compared to their peers (about 3.7 volts). This is the primary reason why Lithium-ion batteries are used in high power applications like EVs.
2. Nickel
Nickel is a crucial element in a lithium-ion battery. Even before Lithium-based batteries, Nickel had found its application in nickel-cadmium and nickel-metal hydride batteries. Hence there is no doubt about having Nickel in Li-ion batteries.
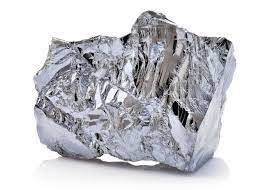
The main reason why people opt for Nickel is that it improves the specific energy and energy density making the battery light and compact. On top of that, it is cheaper and helps for the long life of the battery.
Because of these reasons, Nickel-based batteries have gained more popularity among electric vehicles. Analysts predict that the usage of these batteries is going to grow. Adding more Nickel is going to improve the properties. However, on the flip side, we can accommodate a very less amount of cobalt. This means the battery becomes more unstable and leads to combustion or thermal runaway.
Let me explain briefly about cobalt in the next subsection.
3. Cobalt
Just like nickel, cobalt plays a pivotal role in stabilizing the battery. In fact, as I said above, adding more nickel reduces the stability of the battery. I could say that cobalt compliments nickel.
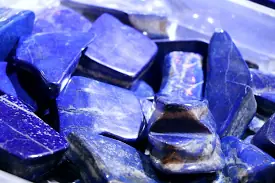
What exactly happens is whenever there is a chemical reaction within the cell, cobalt within the battery reduces and reacts with the excess oxygen by forming Lithium Cobalt Oxide. Also, it promotes higher energy delivery of Lithium ions.
With lesser oxygen that is free, can we say that we have a lesser chance of cathode corrosion. If left unnoticed, the electrodes lose contact hence reducing the performance and efficiency of the battery. This leads to thermal runaway and the excess oxygen within the cell can lead to combustion. Especially if more nickel is present, more is the hazard.
Cobalt is a very expensive metal and this is one of the main contributors to the overall price of the battery. And cobalt mining has a darker side too. Mining cobalt is extremely difficult and has led to human rights abuse and child labour. The labours don’t have a healthy working environment and this is raising concerns. Wion has explained it better than I would. Check out the video attached to know more about this.
Because of the above reason, automakers are trying to shift their cobalt dependency. They have taken two approaches, recycling the metal effectively and reusing it. Secondly, they are looking for other alternatives such as manganese and aluminium. More on it in the next subsection.
4. Manganese And Aluminium
Manganese and aluminium seem to be very promising substitutes for cobalt. It fairly helps in corrosion prevention and is much cheaper. On top of that, mining aluminium and manganese is relatively easy and cause no labour exploitation.
Starting with Manganese, Lithium and Manganese have an amazing bonding relationship. These both can be combined to form a spinel structure. The three-dimensional structure improves the ionic (Lithium) flow within the electrode. Because of this reason, internal resistance within the cathode takes a dive. Hence improving the properties like specific energy, power and thermal stability. However, the cycle of life is affected.
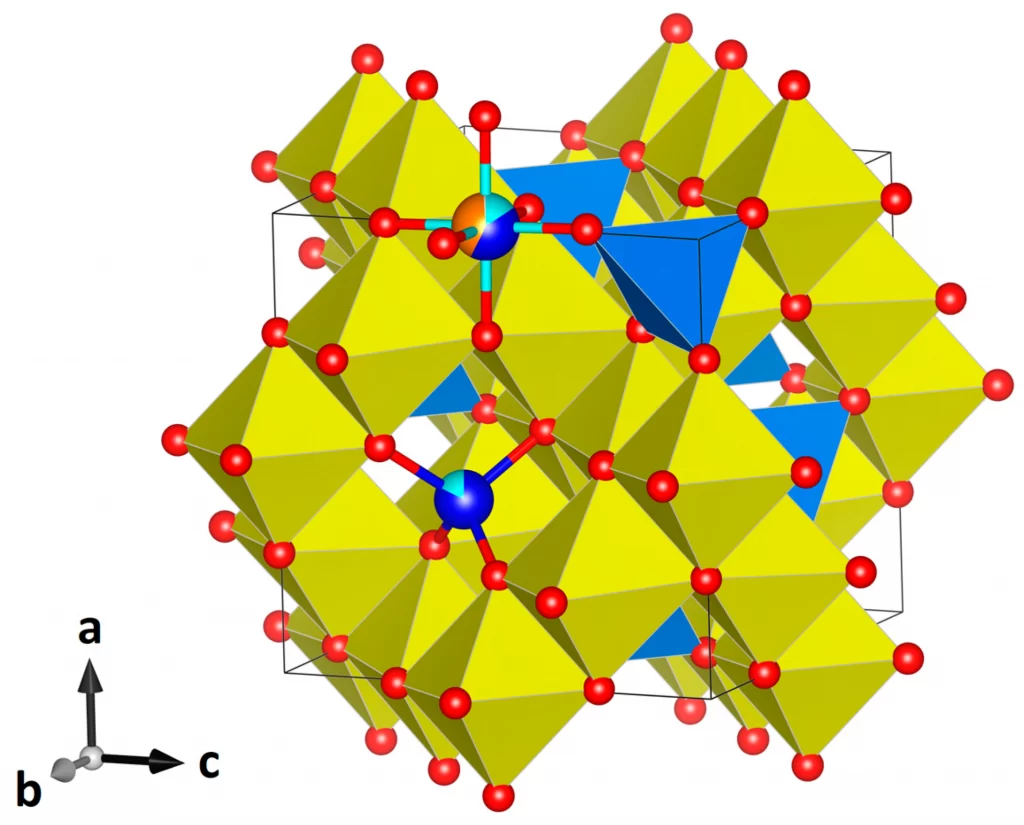
On the other hand, Aluminium is not as good as Manganese. Hence one may not expect great improvement in the battery properties. But the biggest factor that makes it popular is pricing. Hence it is more predominantly popular among non-high-performance applications.
Cobalt is not completely eradicated. At least not among the popular types of lithium-ion batteries like NCA (Nickel Cobalt Aluminium) and NMC (Nickel Cobalt Manganese). The main goal of researchers is to completely chop cobalt off this equation. On the other hand, LFP (Lithium Iron Phosphate) batteries are also adapted. More on this in the upcoming subsection.
Types Of Lithium-ion Batteries
I have walked you through the key elements used in different types of Lithium-ion Batteries, It is now the time to look into the types. There could be a lot more types than I would mention here. But I would strictly limit to batteries with automotive applications or the ones that are popular.
1. Lithium Cobalt Oxide
Lithium Cobalt Oxide (LCO) is the most common type of lithium-ion battery. However, this could not find a spot in the auto sector because of its instabilities. LCO batteries have great specific energy but terrible specific power. Simplifying it, LCO can pack more energy per kilogram but you cannot draw the energy as fast as you can do in a high-specific power battery. On in simple words, do not expect to get pinned to your seat when you smash the throttle.
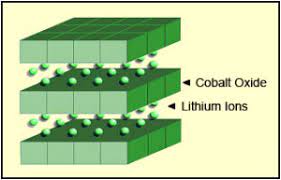
The following is the chemical reaction involved in the LCO battery.
Cathode: Lithium Cobalt Oxide
LiCoO2 <–> CoO2 + x Li+ + x e–
Anode: Carbon (Graphite)
x Li+ + x e– + x C6 <–> x LiC6
Overall reaction:
LiCoO2 + C6 <–> CoO2 + LiC6
As I already told you, they are useful in low-power consumption devices predominantly portable gadgets. Smartphones, laptops, speakers, wearable tech etc use LCO batteries.
2. Lithium Phosphate Battery
Lithium-ion phosphate AKA LFP battery is one of the safest lithium-based batteries currently. The key factor here is that it does not use elements like cobalt and nickel. Hence there is no human rights abuse involved.
On top of that, this battery is a great package with a wide amount of advantages. Starting with the chemistry, the elements that build LFP batteries possess very low internal resistance. This means there will be great thermal stability and high power discharge capabilities. That is why LFPs are deployed in EVs, especially after the whole cobalt issue.
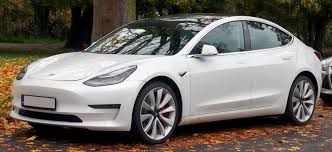
equipped with LFP batteries
With a terminal voltage of 3.2 V, it finds a great replacement for lead-acid batteries. Connect 4 of these cells in series and we get a terminal voltage of 12.8 V which is within the territory of stater motors deployed in passenger vehicles. With great thermal stability and long cycle life, they make a good fit for hybrids too.
The following is the chemical reaction,
Cathode: Lithium Iron Phosphate
LiFePO4 <–> Li+ +FePO4 + e–
Anode: Carbon (Graphite)
Li+ + e– + C6 <–> LiC6
Overall reaction:
LiFePO4 + C6 <–> FePO4 + LiC6
However, in the absence of nickel, they have one of the worst specific energy figures around 90-120 Wh/kg. This means they are not a good fit for the so-called ‘Long range’ version of EVs. This is why companies like Tesla have adapted LFP batteries for their standard-range vehicles while keeping nickel and cobalt-based batteries for long-range variants.
Although these batteries have high thermal runaway figures, they perform poorly in low-temperature conditions. Topping this with one of the highest self-discharge rates among all types of lithium-ion batteries restricts the application mainly for energy storage
3. Lithium Nickel Manganese Cobalt Oxide
I could say that Lithium Nickel Manganese Cobalt Oxide (NMC) is an all-rounder battery. It has a decent specific energy of 150-220 Wh/kg and a reasonable lifespan.
Using manganese reduces the usage of cobalt as manganese does the stabilizing job reasonably well. It forms a stable structure combined with Lithium and helps in reducing the internal resistance of the battery. With a specific power that is above average, NMC batteries have found application in the EV and portable power tool spaces.
Following is the chemical reaction involved in NMC batteries,
Cathode: Lithium Nickel Manganease Cobalt Oxide
LiNiMnCoO2 <–> Li+ + NiMnCoO2 + e–
Anode: Carbon (Graphite)
Li+ + e– + C6 <–> LiC6
Overall reaction:
LiNiMnCoO2 + C6 <–> NiMnCoO2 + LiC6
4. Lithium Nickel Cobalt Aluminium Oxide
Lithium Nickel Cobalt Aluminium Oxide (NCA) batteries are very similar to the NCM ones discussed above. Similar to NCM, NCA is being deployed to reduce the usage of cobalt.
Although both remain almost in the same zone when it comes to lifespan, specific energy and power, they lack safety. Manganese and Nickel do form a more stable bond and hence reduce the chances of thermal runaway.
But the best selling point of these batteries is that it is comparatively cheaper than NCM batteries. Hence, more manufacturers are looking into this chemistry.
The following is the chemistry involved in NCA batteries,
Cathode: Lithium Nickel Cobalt Aluminium Oxide
LiNiCoAlO2 <–> Li+ + NiCoAlO2 + e–
Anode: Carbon (Graphite)
Li+ + e– + C6 <–> LiC6
Overall reaction:
LiNiCoAlO2 + C6 <–> NiCoAlO2 + LiC6
5. Lithium Manganese Oxide
As I already discussed, Lithium and Manganese combine to form a stable spinel bond that improves the characteristics of the battery. Lithium Manganese Oxide (LMO) completely eradicates cobalt and nickel dependency. This not just means lesser human rights violations but also the price of the battery goes down several folds. We can achieve this by retaining the key properties of the battery.
The design of the LMO battery also provides some flexibility. Therefore, manufacturers can optimize between maximizing specific energy, specific power and cycle life. Speaking of cycle life, these batteries do not process good cycle life, unlike the competition.
Following is the chemical reaction involved,
Cathode: Lithium Manganease Oxide
LiMn2O4 <–> Li+ + 2 MnO2 + e–
Anode: Carbon (Graphite)
Li+ + e– + C6 <–> LiC6
Overall reaction:
LiMn2O4 + C6 <–> 2 MnO2+ LiC6
With flexibility in design, these battery finds application with a different state of tune depending on the requirement.
6. Lithium Titanate
Lithium Titanate (LTO) is a rare type of lithium-ion battery. But I wanted to include this in this list because of its performance in niche segments. These batteries have a superior cycle life and are immune to low temperatures. So applications involving super-low temperatures rely on this chemistry.
Unlike the other types of lithium-ion batteries discussed above, LTO replaces the graphite anode and the cathode most likely will be NMC. A key property of this anode material is the fact that there will be no solid electrolyte interphase (SEI) layer formation. More about this in the upcoming section. Just know that this property enables significantly superior life cycles.
The following is the chemical reaction involved,
Cathode: Any of the above-mentioned materials
(LiMn2O4 <–> Li+ + 2 MnO2 + e–) X 3
Anode: Lithium Titanate
3 Li+ + 3 e– + Li4Ti5O12 <–> Li7Ti5O12
Overall reaction:
LiMn2O4 + Li4Ti5O12 <–> 2 MnO2+ Li7Ti5O12
LTO has been quoted as one of the safest batteries on the market. Topping it with low-temperature immunity and high cycle life, LTO finds a lot of applications. Ranging from EVs for cold environments, and street lights to domestic and industrial applications. High prices and terrible specific energy are the only deal-breaker.
Solid Electrolyte Interphase
Solid Electrolyte interphase AKA SEI is a very common phenomenon among different types of Lithium-ion batteries. To be clear it tends to be undesirable as it reduces the battery cycle life greatly. Let me explain how.
SEI happens on the anode in an electrochemical cell while interacting with the electrolytic interface. The mechanism of a battery is when discharging, the lithium ions come out of the cathode and combine with the electrolyte.
On the other terminal, the anode is plated with materials from the electrolyte. It is an organic compound when we speak about lithium-ion batteries. This is not a positive phenomenon as more plating on the anode leads to lesser efficiency. The more the plating, the less will be the electron-ion interaction and hence less will be the performance and cycle life.
To make it worse, these SEI compounds are non-soluble in organic solvents making the entire process irreversible. Thus the battery loses its cycle life over time. This is the reason why LTO batteries have a jaw-dropping cycle life.
Verdict
I have summarised the key attributes of the types of lithium-ion batteries that I have discussed.
Battery | Cathode | Anode | Specific Energy (Wh/kg) | Cycle Life | Terminal Voltage (V) |
Lithium Cobalt Oxide (LCO) | LiCoO2 | Graphite | 150-200 | 500-1000 | 3.6 |
Lithium Iron Phosphate (LFP) | LiFePO4 | Graphite | 90-120 | 2000+ | 3.2 |
NMC | LiNiMnCoO2 | Graphite | 150-220 | 1500-2000 | 3.6 |
NCA | LiNiCoAlO2 | Graphite | 200-260 | 1500-2000 | 3.6 |
Lithium Manganese Oxide (LMO) | LiMn2O4 | Graphite | 100-150 | 300-700 | 3.7 |
Lithium Titanate (LTO) | Any of the above | Lithium Titanate | 50-80 | 3000-7000 | 2.4 |
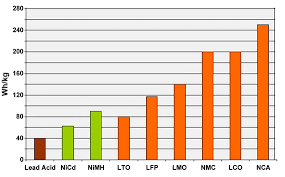
While there is certainly no clear winner from the above list, eliminating cobalt and nickel is pivotal. That not just suppresses the human abuse but also brings the price of the battery down significantly.
If you would ask me, I would suggest going for LFPs when we need decent performance coupled with good safety, and cycle life at a reasonable price. NCA or NCM can be a good choice if we need good specific energy, pay more and opt for NCM if safety is a concern.
Although LTO is a poor performer on paper, its immunity to cycle life and low-temperature working make it a monopoly while dealing with such applications.
If you had made it this far, thanks for your support and keep supporting. This article has enlightened me with a lot of new concepts in battery technology and I would like to give a credit to an article published in batteryuniversity which I referred to a lot for this article.